DNA forms and RNA structure
Last update on the 25th of September, 2017Here we observe three DNA forms and tRNA, calculate torsion angles and analyze interactions of bases and their pairs. Initial assay was made with command-line 3DNA set of programs. Obtained files made possible visualization with Jmol and take snapshots. Further analysis was made with LibreOffice Calc spreadsheet software. Cytosine was drawn in MarvinSketch.
File | Link |
---|---|
A-DNA | gatc-a.pdb |
B-DNA | gatc-b.pdb |
Z-DNA | gatc-z.pdb |
tRNA compared to DNA by torsion angles | 1qrt_torsion.ods |
tRNA and DNA torsion angles | deformed_dna_trna.ods |
DNA forms
gatc-a.pdb, gatc-b.pdb, gatc-z.pdb
With 3DNA fiber
program sample models of A-, B- and Z-DNA were generated in PDB format.
In all three forms major and minor grooves were noticed taking into account depth and width of grooves.
Models are shown in figure 1.

Then, in B-form deoxycytidine 16:A (in PDB notion) was highlighted and inspected which atoms are turned to the major groove and which — to the minor groove. A 3D-model is presented in figure 2.

In total, atoms c16.n1, c16.c2, c16.o2 are turned to the minor groove and atoms c16.c4, c16.n4, c16.c5, c16.c6 are turned to the major groove. The orientation of c16.n3 cannot be unequivocally determined (see the representation of colored base, fig. 3).

With Jmol measurement tools main spiral properties of DNAs were spotted (table 1). It turned out that in A-form major groove is narrower then minor one, though it is still deeper. The orientation of deoxycytidine atoms remains the same.
Prop|Form | A | B | Z |
---|---|---|---|
Spiral type | Right | Right | Left |
Step, Å | 28.03 | 33.75 | 43. 5 |
Bases per coil | 11 | 10 | 12 |
Major groove width, Å | 7.98 5:A.P — 30:B.P 4:A.P — 31:B.P |
17.91 8:A.P — 29:B.P 9:A.P — 28:B.P |
15.17 14:A.P — 27:B.P 13:A.P — 28:B.P |
Minor groove width, Å | 16.97 31:B.P — 13:A.P 30:B.P — 14:A.P |
11.69 34:B.P — 11:A.P 35:B.P — 10:A.P |
9.87 38:B.P — 7:A.P 37:B.P — 8:A.P |
Torsion angles
1qrt_torsion.ods, deformed_dna_trna.ods
Objects of study in this section are tRNA from
1QRT
PDB entry and DNA from 1KSX
entry. The PDB files were converted to the old format with remediator
program and analyzed
with find_pair (-t) filename stdout | analyze
pipeline. Several files were generated. First, the
information from *.out
files was used.
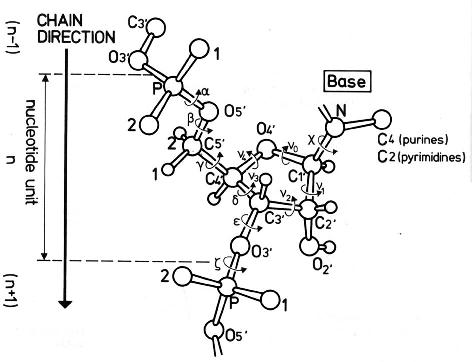
The first property of interest is which form of DNA tRNA is similar to. That was determined with torsion angles
study (see fig. 4). To do that, the values of torsion angles were retrieved from
*.out files
(all DNA models gatc-* were also put through the mentioned pipeline).
As the pipeline calculates angles for only those nucleotides that are engaged in base pairs, the comparison
seems probable. Retrieved values were put into spreadsheet software and were handled with following analysis:
absolute differences of DNA and RNA angle values were taken, averages and standard deviations were calculated;
the lower average means greater similarity of tRNA to a particular DNA form, the lower standard deviation means
bigger consistence of particular angle value in tRNA (as DNA values are the same except Z-DNA). It should be
meant that greater standard deviation means lower applicability of this test or wrong order of Z-DNA angles.
First issue is solved as it is the only reasonable test for this task, second issue is solved with observation that
tRNA angles do not alter (sign too) as Z-form ones. Results are given in the table 2; all raw data is stored in
1qrt_torsion.ods
.
Angle | Strand | Criterion | A | B | Z | Verdict |
---|---|---|---|---|---|---|
α | 1 | average | 56,86 | 67,96 | 106,69 | A |
stdev | 67,88 | 55,74 | 78,16 | A | ||
2 | average | 48,23 | 61,01 | 99,40 | A | |
stdev | 60,37 | 50,06 | 54,69 | A | ||
β | 1 | average | 155,24 | 146,28 | 133,40 | B* |
stdev | 157,74 | 131,03 | 144,04 | B | ||
2 | average | 154,44 | 140,79 | 146,10 | B | |
stdev | 155,43 | 133,52 | 140,58 | B | ||
γ | 1 | average | 45,44 | 51,99 | 132,93 | A |
stdev | 61,38 | 58,32 | 102,42 | A | ||
2 | average | 35,59 | 43,07 | 135,17 | A | |
stdev | 48,99 | 46,06 | 114,62 | A | ||
δ | 1 | average | 6,22 | 58,47 | 31,86 | A |
stdev | 8,68 | 9,01 | 20,92 | A | ||
2 | average | 6,24 | 58,09 | 30,31 | A | |
stdev | 5,75 | 5,88 | 20,95 | A | ||
ε | 1 | average | 39,69 | 39,83 | 60,67 | A|B |
stdev | 75,91 | 73,47 | 57,44 | A|B | ||
2 | average | 31,81 | 34,68 | 61,41 | A | |
stdev | 63,98 | 60,56 | 41,75 | A | ||
ζ | 1 | average | 24,83 | 94,06 | 87,62 | A |
stdev | 42,07 | 45,92 | 81,87 | A | ||
2 | average | 35,39 | 103,34 | 75,33 | A | |
stdev | 57,90 | 65,44 | 74,64 | A | ||
χ | 1 | average | 44,83 | 82,32 | 136,75 | A |
stdev | 99,33 | 67,67 | 109,49 | A | ||
2 | average | 57,30 | 84,87 | 128,70 | A | |
stdev | 107,89 | 74,12 | 108,47 | A |
Despite huge values, it seems possible to say that tRNA is more similar to A-DNA than to other forms.
The second point of interest are average values of torsion angles in sample tRNA and DNA. They were
calculated in deformed_dna_trna.ods
spreadsheet. Also, the most deformed nucleotides
were determined with rank test. First, the absolute differences of each base angle and average were
calculated. Then, each base for each angle were ranked from the biggest to the smallest difference.
Ranks were summed for each base and, finally, sums were ranked from the lowest to the biggest.
Since then, the base with "1" rank is the most deformed. Such test were made for all 4 strands (see table 3).
Angle|Strand | DNA1 | DNA2 | tRNA1 | tRNA2 |
---|---|---|---|---|
α | -20,52 | -20,51 | -40,59 | -44,78 |
β | 31,41 | 31,40 | 22,39 | 12,07 |
γ | 20,76 | 20,74 | 53,30 | 51,66 |
δ | 140,19 | 140,19 | 85,31 | 85,18 |
ε | -136,21 | -136,22 | -118,15 | -125,43 |
ζ | -88,04 | -88,03 | -63,15 | -53,57 |
χ | -116,43 | -116,44 | -121,76 | -116,62 |
D | 27T (12:K) | 28T (12:O) | 10G (52:B) | 7G (65:B) |
DNA average values are more consistent between strands than tRNA ones. This can be explained as DNA usually takes regular double-strand structure, wereas tRNA double-strand structure is variable which leads to unique abilities of its tertiary structure. There is no reason in showing the most deformed nucleotides as it would be llittle use of comparing them to "normal" nucleotides (which also must be determined).
tRNA stems and base pairs

Stems are those elements of RNA secondary structure, which look similar to DNA in terms of connection: consequative nucleotides are connected with hydrogen bonds to the other strand. In given tRNA 4 stems were identified (fig. 5). As tRNA plays a highly conserved role, it stems are named due to their functional or sequence features.
Hydrogen bonds in stems are not the only type of connection between nucleotydes which sustain tRNA secondary and tertiary structures. Those for particular tRNA are given in figure 6.
12 (0.007) ....>B:..54_:[..U]U-**--A[..A]:..58_:B<.... (0.003) | 13 (0.010) ....>B:..55_:[..U]U-**+-G[..G]:..18_:B<.... (0.010) x 25 (0.004) ....>B:..13_:[..A]A-**+-A[..A]:..45_:B<.... (0.006) | 26 (0.004) ....>B:..14_:[..A]A-**--A[..A]:..21_:B<.... (0.008) | 27 (0.006) ....>B:..15_:[..G]G-**+-C[..C]:..48_:B<.... (0.011) x 28 (0.014) ....>B:..19_:[..G]G-----C[..C]:..56_:B<.... (0.005) + 29 (0.003) ....>B:..46_:[..U]U-**+-U[..U]:..47_:B<.... (0.007) +
tRNA is rich with non-Watson-Crick base pairs. All of them are shown in figure 7A.
12 (0.007) ....>B:..54_:[..U]U-**--A[..A]:..58_:B<.... (0.003) | 13 (0.010) ....>B:..55_:[..U]U-**+-G[..G]:..18_:B<.... (0.010) x 14 (0.009) ....>B:..37_:[..A]A-**--U[..U]:..33_:B<.... (0.004) | 15 (0.007) ....>B:..38_:[..U]U-**--U[..U]:..32_:B<.... (0.005) | 21 (0.005) ....>B:..44_:[..C]C-**--A[..A]:..26_:B<.... (0.005) | 25 (0.004) ....>B:..13_:[..A]A-**+-A[..A]:..45_:B<.... (0.006) | 26 (0.004) ....>B:..14_:[..A]A-**--A[..A]:..21_:B<.... (0.008) | 27 (0.006) ....>B:..15_:[..G]G-**+-C[..C]:..48_:B<.... (0.011) x 29 (0.003) ....>B:..46_:[..U]U-**+-U[..U]:..47_:B<.... (0.007) +
Three non-canonical base pairs (12, 14 and 27) are comprised of "canonical" bases. However, interaction are non-Watson-Crick, which is shown in fig. 7B.

Putative stacking interactions
Stacking is also the interaction which builds nucleic acid structure. 3DNA allows to quantify square of
overlap area of two consequative base pairs. The most and the least stacked pairs were found, images
were obtained with ex_str
and stack2img
programs. Also, the position of
pairs was observed in Jmol. Figure 8 presents the composite picture.

As it is seen, the most stacked pairs are indeed placed close and overlap hugely. The least stacked pairs are placed relatively far from each other and not parallel.